Simulation of Laser Wakefield Acceleration
In 2004, researchers at the U.S Department of Energy’s Lawrence Berkeley National Laboratory took a giant step toward realizing the promise of laser wakefield acceleration, by guiding and controlling extremely intense laser beams over greater distances than ever before to produce high-quality energetic electron beams. The experimental results were then analyzed by running the VORPAL (known today as VSim) plasma simulation code on supercomputers at DOE’s National Energy Research Scientific Computing Center (NERSC) at Berkeley Lab. The results were published in the Sept. 30 issue of Nature magazine, which chose an image from the NERSC simulations for the magazine’s cover.
For over 25 years physicists have been trying to push charged particles to high energies with devices called laser wakefield accelerators. In theory, particles accelerated by the electric fields of laser-driven waves of plasma could reach, in just a few short meters, the high energies attained by miles long machines using conventional radio frequency acceleration. Stanford’s linear accelerator, for example, is two miles long and can accelerate electrons to 50 GeV (50 billion electron volts). Laser wakefield technology offers the possibility of a compact, high-energy accelerator that can be used for probing the subatomic world, studying new materials and new technologies, as well as applications in the health care field. In plasmas, researchers have generated electric fields a thousand to ten thousand times greater than in conventional accelerators. Unfortunately these large fields exist only over the short distance that a laser pulse remains intense and for tightly focused beams, that distance is typically only a few hundred micrometers. The resulting beams are of relatively poor quality, with particle energies so widespread that fewer than one percent have enough energy for use in scientific applications.
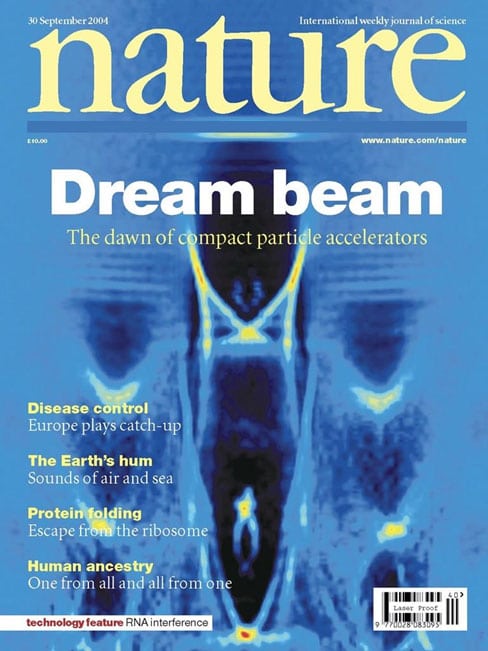
The Berkeley Lab researchers, led by Wim Leemans of the Center for Beam Physics in Berkeley Lab’s Accelerator and Fusion Research Division, achieved high-quality beams by first shaping a channel through hydrogen gas with powerful, precisely timed laser pulses, then accelerating electron bunches through the plasma inside the channel. Because of the controlled accelerator length and the characteristics of the channel, there are several billion electrons in each bunch within a few percent of the same high energy of more than 80 MeV.
“Laser wakefield acceleration works on the principle that by sending a laser pulse through a gas to create a plasma-separating negatively charged electrons from positively charged ions in the gas — some of the free electrons will be carried along in the wake of the plasma wave created by the laser,” Leemans explains. “Imagine that the plasma is the ocean, and the laser pulse is a ship moving through it. The electrons are surfers riding the wave created by the ship’s wake.”
To analyze their successful experiment, the group collaborated with John R. Cary and the team at Tech-X Corporation in Boulder, Colorado, using the VORPAL plasma simulation code (known today as VSim) to model their results on supercomputers at NERSC. This modeling allowed the scientists to see the details of the evolution of the experiment.
“With VSim, one can see the laser pulse breakup and the injection of particles into the laser-plasma accelerator,” said Cary, CEO of Tech-X Corp. and a professor of physics at the University of Colorado. “This allows one to understand how the injection and acceleration occur in detail so that the experiment’s designers can figure out how to optimize the process.”
Simulation by Peter Messmer
By tailoring the plasma channel conditions and laser parameters, the researchers are first able to achieve clean guiding of laser beams of unprecedented high intensity, while suppressing electron capture. This paves the way for using laser-powered plasma channels as ultra-high-gradient accelerating structures. Next, by using even higher peak powers, plasma waves are excited that are capable of picking up background plasma electrons, rapidly accelerating them in the wake’s electric field, then finally subsiding just as the surfing electrons reach the dephasing length, when they are on the verge of outrunning the wake.
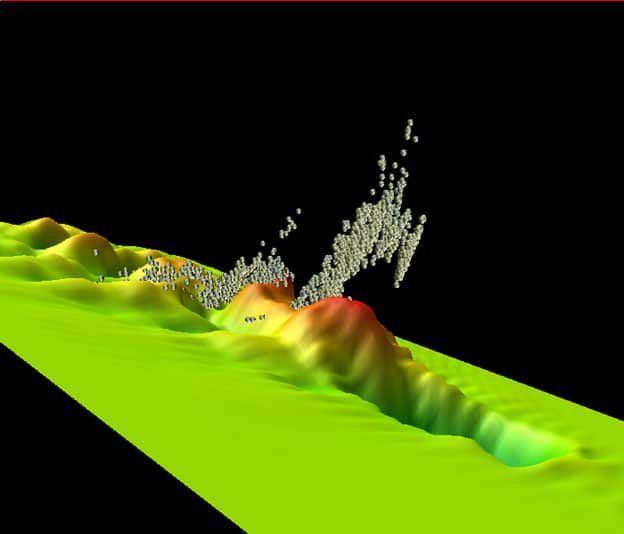
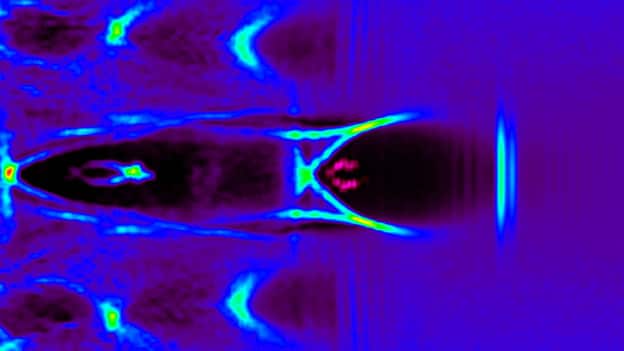
Simulation by Cameron Geddes, LBNL and Tech-X Corporation
Next, the researchers needed to determine how the electrons in the bunch are accelerated to nearly the same energy. Following the experiments, the Berkeley Lab researchers, along with their collaborators at Tech-X, used VSim to model the evolution of the high-quality electron bunches on supercomputers at NERSC. They found that the formation of large bunches of electrons with nearly the same high energy is due to several factors. Most critical is matching the long acceleration path to the dephasing length. Other factors include plasma density and laser power, and how the laser pulse evolved during its propagation in the plasma channel.
Article Credit:
NERSC News, October 2004
Video Credit:
VSim visualization by Peter Messmer
Different incarnations of laser-wakefield accelerators that show the background electron density (surface) plus some high-energy particles (beam) as particles.